- Home
- Teaching & Learning
- General
- How understanding the brain can help your teaching
How understanding the brain can help your teaching
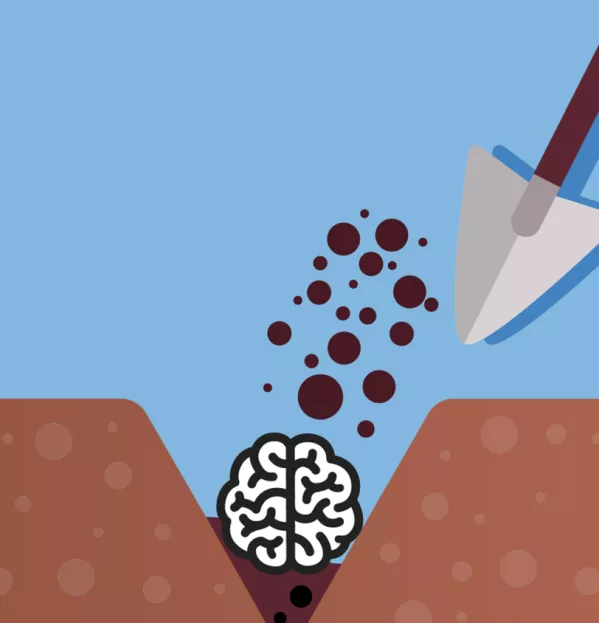
Paulo is a born athlete. Doing a backflip comes as naturally as breathing to him - he jumps up and balls his legs up toward his chest. Voilà - he flips right over and lands, neat as you please, on his feet. Baseball? Football? No problem. He’s a star.
Even learning to ride a bike came easily to Paulo. Five years earlier, he’d watched his big brother, Robert, teetering toward stability on the new bike their mum had scraped to buy. Then, when Robert went in for lunch, Paulo hopped on the far-too-large-for-him bicycle and zipped off to join his friends as if he’d been cycling all his life.
But here’s the important point: both Paulo and Robert learned to ride a bike. And Robert, even though he was slower and took more falls as he was learning, never told himself “I guess I just don’t have the biking gene”. In fact, even though learning to ride resulted in skinned knees and elbows, and a lot of nerve-racking moments, Robert kept on through the pain until he got it.
Why does it seem natural that students might believe they can become good at riding a bike - even though they learn at different speeds and it’s sometimes painful - but in school, when students might learn certain subjects more slowly and with more difficulty than others, they give up on themselves, believing they don’t have a talent for a particular subject?
There are other mysteries to learning. Put babies around native speakers of a language, and the language will be absorbed with ease. Put babies around a bunch of books and you may find chewed corners, but they won’t know how to read. Why do many students struggle with reading when they seem to absorb their native language like a sponge? And why do students often struggle when it comes to maths?
These are questions that we have explored in our new book, Uncommon Sense Teaching - and they are questions that it pays to think about now, as teachers around the world are grappling with how best to support students in the aftermath of a pandemic. Understanding how the brain has evolved to learn, and what that means for effective teaching, has perhaps never been more important.
To reach that understanding, we need to explore two issues. First, why is some information, such as recognising a human face or speaking a first language, easier to learn than other information for virtually all students? Second, why are some subjects, such as reading and maths, harder to learn for some students than others? Why can a six-year-old without access to an education system learn her native language with ease, but be unable to learn to read or do maths without plenty of guided instruction?
To understand how learning takes place, we should first give you a sense of how human brains change and grow as babies develop into adults. In early infancy, neurons (nerve cells) are like migratory birds. They are born and then shift toward their final neural homes, where they form structures that extend out to connect with other neurons. Rich nests called neuropils, which are filled with intertwined neurons, form. The growing neuropils provide a bounteous crop of new connections (synapses) between neurons. All this growth and activity peaks at around 2 years of age.
The hard stuff
But then, like the onset of winter, pruning sets in. As children move toward adolescence, parts of the neurons are snipped away, decreasing the number of synapses. During this process, a child’s environment matters. A healthy, varied environment allows more of the neural connections to stay intact. A restricted, stressful environment can cause too much pruning - like plants that have had most of their foliage sheared off.
Your vision and hearing are processed in the back of your brain. This area matures first, in early childhood. Mental maturation - which means pruning and loss of flexibility - gradually moves toward the front of the brain. The very last area to mature is the prefrontal cortex, where planning and judgement take place. (This explains why secondary school students sometimes behave in surprisingly immature ways.) The ability to adjust the brain’s connections doesn’t stop at maturity, however. New synaptic connections, as well as pruning, continue throughout people’s lifetimes.
As we’ve mentioned, babies quickly learn to recognise faces, even though this feat is so difficult that it took many decades of intense research for facial recognition algorithms to come into being.
Likewise, babies can pick up their first language quickly and naturally. Around the child’s first year, their vocabulary learning begins to accelerate through a fast-mapping process, where a toddler needs to hear a word only a few times before she’s got it. Between 20 and 24 months of age, researchers estimate that children suddenly triple their productive vocabularies. All this while also assimilating the syntactic structure!
So, why are some things easier for us to learn than others? Cognitive developmental and evolutionary psychologist David Geary has put forwards a theory (as previously written about in Tes) to explain what is happening here.
Recognising faces and learning how to speak a first language - what we might call the easy stuff - Geary has termed “biologically primary” material.
Our brains naturally learn this type of information. Neurons seem to link together as if by magic, even though it’s magic honed by thousands of generations of evolutionary selection. Babies with the neural architecture to recognise and speak with those around them survived. Those who couldn’t died.
In contrast with the easy stuff is what we’ll call hard stuff - that is, what Geary terms “biologically secondary” material. Those are skills and abilities that our species hasn’t evolved to do - even though by the time we might reach adulthood and have had plenty of practice, they might seem easy. We certainly didn’t evolve to casually grab our laptop to scan news headlines or to work out our taxes using sophisticated maths programs. And our students do not naturally know how to solve for x or use a semicolon correctly. To do these and other types of activities expected of a responsible adult, we have to go through years of specialised training in reading, writing and complex maths, not to mention geography, politics, economics and history.
We might not have evolved to do these things, but evolution still plays a role in learning biologically secondary material. Evolution involves a constant process of developing tools, including teeth, fins, hooves and beaks. It’s a bit like gambling: with each new generation, there’s a random throw of the genetic dice, and critters with the tiniest improvements in their tools are more likely to win - which means they get to pass their genes down to the next generation. Brains, of course, are part of those tools. Evolution has selected and shaped brains just as it has everything else.
While evolution was winnowing tools and brains, one type of brain - that of humans - developed a particularly flexible multipurpose cognitive processing ability. By repurposing neural circuits that were originally developed for other uses, human brains can accomplish feats that were undreamed of in previous evolutionary times. For example, as we’ll see, the “recognising the face” part of the brain can be repurposed to allow us to learn to read.
Saying ‘I do’
In other words, to learn hard stuff - secondary materials - our brains have to stretch and rewire in ways they have not had to do in the previous eras of human evolution. The neuronal recycling hypothesis, developed by leading neuroscientist Stanislas Dehaene, independently comes to similar conclusions.
Abilities such as reading and maths involve reconfiguring parts of the brain commonly used for other purposes. But Dehaene’s conclusions go a step further. He argues that when the brain does its reconfiguring, it always selects the areas with functions that are the most similar, from an evolutionary perspective, to the new abilities a person is trying to acquire. For instance, letters always seem to invade the occipitotemporal cortex - a part of the brain that ordinarily detects objects and scenes. This happens no matter where or in what culture around the world the person learns to read.
In other words, as Dehaene concludes, although the brain is plastic, it’s not super-plastic - new skills can’t just grow anywhere, but instead are limited to some degree by pre-existing neural connections and anatomical constraints.
So, what does all of this mean for how we teach? Repurposing neural circuits isn’t easy. Learning a new concept or technique can take days or weeks of deliberate practice with corrective feedback. And it can take years to become an expert in, say, mathematics, teaching or medicine. That’s why educational systems have developed around the world - to facilitate the repurposing.
What is the most effective way to rewire students’ brains as they begin grappling with more and more difficult material? Evidence indicates that the more biologically secondary - that is, the tougher - the material, the more direct instruction is needed.
In teacher-directed learning, the teacher leads the lesson and scaffolds instruction until the students master the material. Teachers frequently refer to it as “I do, we do, you do”.
Direct instruction uses the “learn it, link it” approach to teaching. During the “learn it” phase, the teacher presents new concepts or skills (“I do”), and scattered neurons search to make connections. But direct instruction doesn’t stop at the teacher explaining the concept. That would be a simple lecture, which doesn’t form lasting links between neurons (we’ll say more about that later).
Instead, in direct instruction, the teacher explains the new content with different examples and demonstrations, as students follow along and get plenty of chances to practise. During practice, the teacher vigilantly offers corrective feedback (this is the “we do” stage). So, the “learn it” phase encompasses both the “I do” and “we do” stages of direct instruction.
Don’t lecture me
During the “you do” stage, students practise multiple times on their own and are able to demonstrate their understanding independently.
The “link it” phase begins at the “you do” stage of direct instruction, but it doesn’t stop there. Not only do we want students to be able to strengthen their neural links, but we also want them to extend their learning even further. While students practise under a variety of conditions, they add new information and consider different perspectives. In doing so, they are strengthening and extending their neural pathways with additional links. As Geary notes: “Many of these are links that are not naturally very strong, and so for much of secondary learning, a lot of practice distributed over time is needed to make sure the new links stay linked (that is, to be sure the student retains the new knowledge over the long term).”
This “I do, we do, you do” approach scaffolds the instruction of more difficult materials and gradually releases the responsibility of the material over to the student. At first, the teacher takes responsibility for successfully working through the problem, concept or skill. During guided practice (“we do”), she slowly releases that responsibility to the student.
A word of caution about scaffolding: when scaffolds are removed too early, newly learned information isn’t built and solidly reinforced in neo (long-term memory in the neocortex). This is why our students can become frustrated at the kitchen table when they’re doing their homework or why they suddenly freeze during the exam; they needed more time with their teachers in the “we do” stage of direct instruction.
However, once students have demonstrated proficiency with the concept in class under the teacher’s watchful eye, it’s a good idea to assign a few representative problems for homework. Ideally, students complete homework after school or the following day, when several hours have passed. The homework interrupts the forgetting and solidifies the new knowledge.
Direct instruction is effective with all types of learners, but especially novice learners or learners with lesser-capacity working memory. The approach allows students who struggle with attention to attend to the essential ideas in a lesson. Want to teach students how to solve chemical equations? Direct instruction. Want to explain the factors that led to the end of the Cold War? Direct instruction. In either lesson, the teacher takes large pieces of information and breaks them down into understandable parts for students to try to do, or to retrieve and rehearse. Remember, teaching is ultimately about what we get out of students’ heads, not what we try to put in.
This explains why direct instruction is not the same as delivering a lecture. Listening to a presentation may involve intense cognitive processing. The processing can be so intense, in fact, that it doesn’t take long for students to tire and tune out. That’s why listening to a lecture - sometimes described as “chalk and talk” - is called a passive activity. Students might look attentive but not be following at all.
The reality is that learning complex, biologically secondary material is not a spectator sport - it demands that students actively grapple with the material. The grappling can be as simple as reviewing notes with a partner after a five- to seven-minute presentation.
Teachers should also avoid extended passivity by allowing students to watch or listen to lengthy videos or audio texts. While it might seem like a great break for students to watch Hamlet after reading and analysing the play, it can be a waste of precious instructional time.
When segments of video are shown as part of high-quality direct instruction, it’s a good idea to set a purpose for viewing and to provide students with guiding questions (fill-in-the-blank, multiple-choice and short-answer) to complete while watching. It’s also a good idea to pause intermittently to offer additional commentary, clarify any confusing parts and solicit students’ opinions and impressions. As a follow-up, you can have students do something related to the video and what they saw or how it made them feel about their world.
So, direct instruction works best for learning biologically secondary material - and it works in part by incorporating active, not passive, approaches. But what about student-directed approaches to learning? Where does this leave those? Can they ever be useful for teaching difficult material?
Often, teacher-directed instruction and student-directed instruction are viewed as opposing methodologies. But in practice, teacher-directed instruction sets students up for successful student-directed learning. The more difficult (biologically secondary) the material, the more that students need coaching (teacher-directed approaches) to launch them toward independence (student-directed approaches). As students strengthen their neural links, they gain autonomy in their learning.
All too often, student-directed approaches are begun too early when teaching specific materials. Novice learners may find themselves easily frustrated because they are put in situations in which they have to try to teach themselves complex material that they may be only vaguely familiar with. The truth is, when it comes to concepts learned declaratively, students can take over the wheel to drive their own learning only after they understand the rules of the road and can implement proper driving techniques.
Ultimately, there is a time and a place for both direct instruction and student-directed learning. During the “I do” and “we do” stages of direct instruction, students learn it. Once students have demonstrated proficiency on their own, student-directed experiences are appropriate because the material is in the neocortex, even if it’s not quite stable yet.
At this point, students are equipped to link it - that is, strengthen and extend their neural links independently. In this way, the two sides of the continuum work well together. After all, the goal of education is to teach students new information and skills so that they can become independent learners. That remains true even when we are working to help them bounce back from the effects of a pandemic.
Barbara Oakley is a professor of engineering at Oakland University in Rochester, Michigan. Beth Rogowsky is an associate professor at Bloomsburg University of Pennsylvania. Terrence J Sejnowski is the Francis Crick Professor at the Salk Institute for Biological Studies
From Uncommon Sense Teaching by Barbara Oakley PhD, Beth Rogowsky EdD and Terrence J Sejnowski PhD, published by TarcherPerigee, an imprint of Penguin Publishing Group, a division of Penguin Random House LLC. Copyright © 2021 by Barbara Oakley, Beth Rogowsky and Terrence J Sejnowski
This article originally appeared in the 18 June 2021 issue under the headline “Why the evolution of the brain matters to your teaching”
You need a Tes subscription to read this article
Subscribe now to read this article and get other subscriber-only content:
- Unlimited access to all Tes magazine content
- Exclusive subscriber-only stories
- Award-winning email newsletters
Already a subscriber? Log in
You need a subscription to read this article
Subscribe now to read this article and get other subscriber-only content, including:
- Unlimited access to all Tes magazine content
- Exclusive subscriber-only stories
- Award-winning email newsletters
topics in this article